Algorithmic Architecture: Expanding Possibilities in Rhino for Assignment
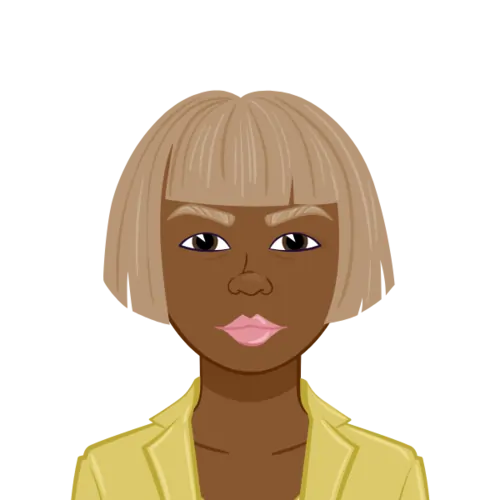
In the ever-evolving landscape of architecture and design, the fusion of computational algorithms and innovative software platforms has ushered in a new era of creative exploration and expression. Within this dynamic milieu, Rhino stands as a stalwart beacon, offering students a gateway to the captivating realm of algorithmic architecture. As the boundaries between the physical and the digital blur, architects and designers are increasingly turning to computational tools to envision, iterate, and realize groundbreaking architectural forms.
At its essence, algorithmic architecture represents a paradigm shift—a departure from traditional design methodologies towards a more fluid and iterative approach. By harnessing the power of algorithms, designers can transcend the constraints of manual drafting and explore a universe of geometric possibilities. Rhino, with its intuitive interface and robust capabilities, serves as the perfect canvas for students to embark on this exhilarating journey of discovery and innovation.
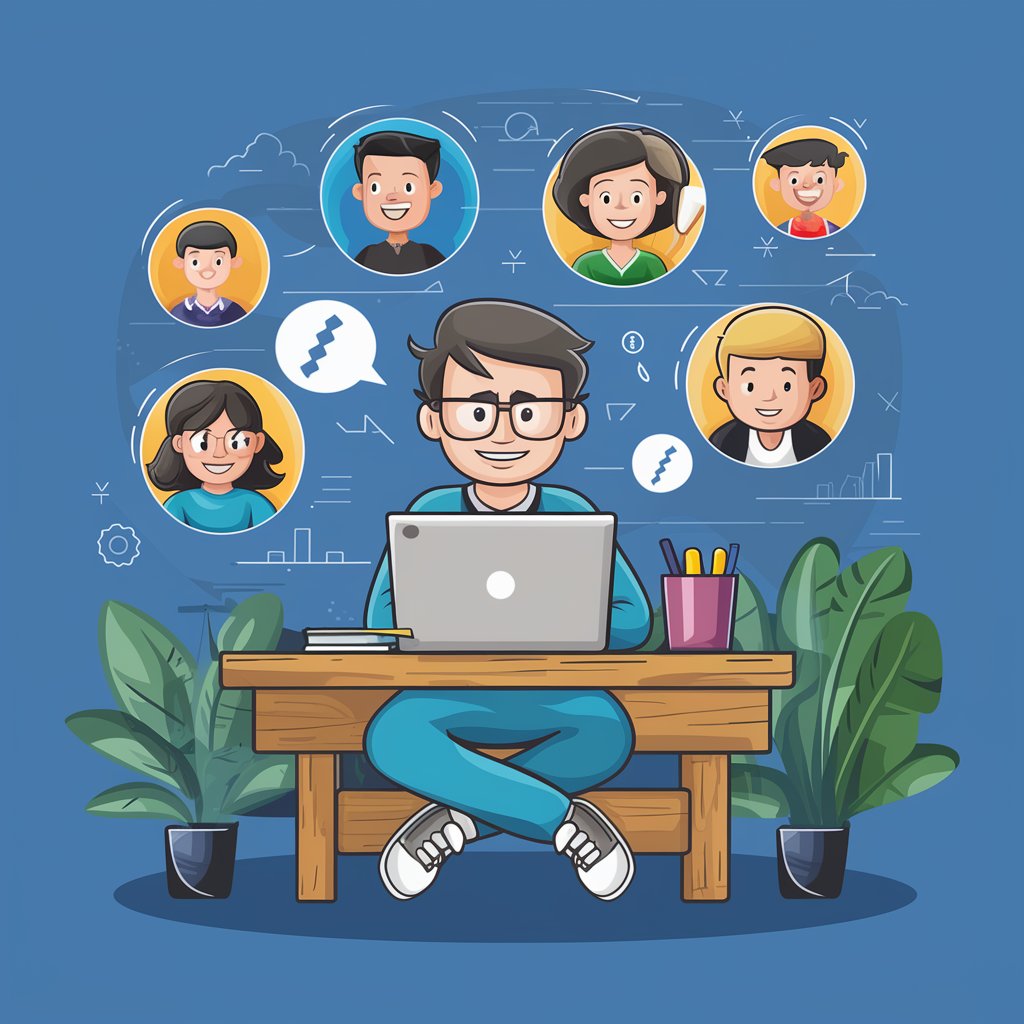
In this blog, we delve deep into the intricate tapestry of algorithmic architecture within Rhino, unraveling its myriad applications and offering insights that can empower students to tackle Rhino assignment, From the fundamentals of parametric modeling to the nuances of generative algorithms, we navigate through the complex terrain of computational design, providing a roadmap for students to unlock their full potential.
Join us as we embark on a quest to unravel the mysteries of algorithmic architecture, where imagination knows no bounds and every line of code holds the promise of architectural innovation. Through exploration, experimentation, and collaboration, students can harness the transformative power of Rhino to shape the future of design and redefine the boundaries of architectural possibility.
Understanding Algorithmic Architecture:
Algorithmic architecture represents a paradigm shift in the way architects conceive, develop, and execute designs. It transcends traditional modes of architectural creation by introducing computational algorithms as fundamental tools for design exploration and realization. At its core, algorithmic architecture embodies a fusion of mathematics, logic, and design principles, enabling architects to generate complex forms, optimize performance parameters, and respond dynamically to contextual factors.
The essence of algorithmic architecture lies in its ability to imbue designs with a sense of generativity and adaptability. By encoding design intent into algorithmic rules and parameters, architects can create systems that produce a multitude of design variations, each uniquely tailored to specific criteria or constraints. This iterative process of exploration and refinement fosters a dynamic dialogue between designer and algorithm, culminating in the emergence of novel architectural expressions that defy conventional categorization.
Within the context of Rhino, algorithmic architecture finds fertile ground for exploration and experimentation. The platform's robust modeling capabilities, coupled with the visual programming environment of Grasshopper, provide students with a sandbox where they can playfully manipulate parameters, iterate through design iterations, and uncover unforeseen design possibilities. From parametric surfaces to intricate spatial configurations, Rhino empowers students to traverse the vast landscape of algorithmic design with confidence and curiosity.
As students delve deeper into the realm of algorithmic architecture, they encounter a rich tapestry of concepts and techniques that underpin its practice. Parametric modeling serves as the cornerstone, enabling designers to define relationships between geometric entities and drive form generation through algorithmic manipulation. Generative algorithms introduce an element of randomness and variation, allowing designers to explore design spaces beyond the confines of their imagination.
Optimization emerges as a critical aspect of algorithmic architecture, as designers seek to fine-tune designs to meet specific performance criteria. Whether optimizing for structural efficiency, energy performance, or user experience, algorithms provide a means to iteratively refine designs and achieve optimal outcomes. Data-driven design further enriches the design process by integrating real-world data, such as site conditions or user preferences, to inform design decisions and generate contextually responsive solutions.
At its essence, algorithmic architecture embodies a philosophy of emergence, wherein complex behaviors and patterns emerge from the interaction of simple components within a computational system. This emergent quality imbues designs with a sense of vitality and dynamism, fostering a deeper connection between architecture and its environment.
In the pursuit of algorithmic architecture assignments, students are encouraged to embrace a spirit of curiosity, experimentation, and collaboration. By immersing themselves in the principles and practices of computational design, students can unlock the full potential of Rhino as a tool for architectural innovation. Through hands-on exploration, critical inquiry, and peer engagement, students can cultivate the skills and insights necessary to navigate the complexities of algorithmic architecture and chart new territories of design possibility.
Rhino: A Gateway to Algorithmic Exploration:
Within the vast landscape of computational design tools, Rhino stands as a beacon of versatility and innovation, offering students a gateway to the captivating realm of algorithmic architecture. Renowned for its intuitive interface, robust modeling capabilities, and extensive plugin ecosystem, Rhino provides an ideal platform for students to embark on a journey of algorithmic exploration and discovery.
At the heart of Rhino's appeal lies its adaptability to a wide range of design tasks and workflows. Whether sculpting organic forms, modeling intricate geometries, or simulating complex assemblies, Rhino's flexible toolkit empowers students to bring their design visions to life with unparalleled precision and efficiency. The platform's support for parametric modeling, coupled with the visual programming environment of Grasshopper, further enhances its appeal as a tool for algorithmic exploration.
Grasshopper, Rhino's visual scripting language, serves as the linchpin of algorithmic exploration within the platform. By enabling students to create and manipulate parametric relationships through a visual interface, Grasshopper democratizes the process of algorithmic design, making it accessible to designers of all skill levels. Through the intuitive manipulation of nodes and connectors, students can experiment with algorithmic rules and parameters, iteratively refining their designs and uncovering new possibilities along the way.
Within the realm of algorithmic architecture, Rhino offers students a canvas where they can translate abstract ideas into tangible architectural expressions. From the generation of parametric patterns to the simulation of structural behaviors, Rhino's robust capabilities empower students to explore a diverse array of design concepts and methodologies. Whether designing for performance-driven outcomes or purely aesthetic considerations, Rhino provides the tools necessary to realize even the most ambitious design visions.
One of Rhino's greatest strengths lies in its extensibility through plugins, which further expand its capabilities and unlock new possibilities for algorithmic exploration. From advanced analysis tools to parametric modeling libraries, Rhino's plugin ecosystem offers students a wealth of resources to enhance their design workflows and push the boundaries of computational creativity. Whether experimenting with generative algorithms, exploring kinetic structures, or integrating environmental simulations, Rhino's plugins provide students with the tools they need to realize their design ambitions.
As students navigate the complexities of algorithmic exploration within Rhino, they are encouraged to approach their assignments with a spirit of curiosity, experimentation, and innovation. By embracing the platform's capabilities and pushing the boundaries of what is possible, students can unlock new avenues of design exploration and chart a course towards architectural innovation. Through hands-on experimentation, peer collaboration, and critical reflection, students can harness the transformative power of Rhino to shape the future of design and redefine the possibilities of algorithmic architecture.
Grasshopper: Unleashing the Power of Parametric Design
At the intersection of creativity and computation lies Grasshopper, the visual scripting language that serves as the catalyst for unleashing the power of parametric design within Rhino. As students venture into the realm of algorithmic architecture, Grasshopper emerges as a potent tool for harnessing the potential of parametric relationships to drive design exploration and innovation.
Grasshopper's intuitive interface and visual programming paradigm lower the barriers to entry for students, enabling them to create complex parametric models without the need for extensive coding knowledge. Through the manipulation of nodes and connectors, students can construct intricate algorithms that encode design intent, allowing for the creation of adaptive and responsive architectural forms.
Parametric design, at its essence, revolves around the concept of defining relationships between design parameters and geometry. Grasshopper empowers students to explore these relationships through a visual and intuitive interface, facilitating an iterative design process where changes to input parameters propagate seamlessly through the model, resulting in dynamic and fluid design iterations.
By breaking down design tasks into discrete components and defining the relationships between them, Grasshopper enables students to explore a wide range of design variations quickly and efficiently. Whether adjusting the dimensions of a parametric facade, exploring different structural configurations, or optimizing for environmental performance, Grasshopper provides students with the tools to iterate through design alternatives rapidly and iteratively.
Furthermore, Grasshopper's integration with Rhino's modeling environment allows for seamless interoperability between parametric and traditional modeling workflows. Students can leverage Grasshopper to drive Rhino's modeling tools parametrically, enabling them to create complex geometries that would be challenging or impossible to achieve through manual manipulation alone.
As students delve deeper into Grasshopper's capabilities, they encounter a rich ecosystem of plugins and libraries that further extend its functionality and unlock new possibilities for parametric design exploration. From advanced simulation tools to parametric optimization algorithms, Grasshopper's plugin ecosystem offers students a wealth of resources to enhance their design workflows and push the boundaries of computational creativity.
In the pursuit of algorithmic architecture assignments, students are encouraged to embrace Grasshopper as a powerful tool for unleashing their creativity and exploring the potential of parametric design. By immersing themselves in the principles and practices of parametric modeling, students can unlock new avenues of design exploration and innovation, paving the way for future success in the dynamic world of computational design. Through experimentation, collaboration, and a willingness to push the boundaries of what is possible, students can harness the transformative power of Grasshopper to shape the future of architecture and redefine the possibilities of parametric design.
Key Concepts in Algorithmic Architecture:
As students delve into the realm of algorithmic architecture, it's essential to grasp the fundamental concepts that underpin this innovative approach to design. These key concepts serve as the building blocks for understanding and implementing algorithmic processes within Rhino and Grasshopper, enabling students to tackle assignments with confidence and creativity. Let's explore these concepts in greater detail:
-
Parametric Modeling:
At the heart of algorithmic architecture lies parametric modeling, a methodology that involves defining geometric entities based on parameters and rules. Parametric modeling allows designers to create flexible and adaptive designs by establishing relationships between design elements and variables. By adjusting parameters, such as dimensions or angles, designers can explore a multitude of design variations quickly and iteratively.
-
Generative Algorithms:
Generative algorithms are computational routines that produce diverse design outcomes based on predefined rules and inputs. These algorithms often leverage randomness or iterative processes to generate a range of design solutions. In algorithmic architecture, generative algorithms enable designers to explore complex design spaces, fostering creativity and innovation through the exploration of unexpected design outcomes.
-
Optimization:
Optimization involves refining designs to meet specific performance criteria or objectives. Within algorithmic architecture, optimization algorithms analyze design parameters and iteratively adjust them to improve performance metrics such as structural efficiency, energy consumption, or spatial quality. By integrating optimization techniques into their workflow, students can create designs that are not only visually compelling but also functionally optimized.
-
Data-Driven Design:
Data-driven design involves using data inputs, such as site conditions, environmental factors, or user preferences, to inform design decisions and generate responsive architectural solutions. In algorithmic architecture, data-driven design techniques enable designers to create designs that are contextually sensitive and adaptive to their surroundings. By integrating data inputs into their algorithms, students can create designs that respond dynamically to changing conditions and user needs.
-
Emergence:
Emergence refers to the phenomenon where complex behaviors or patterns emerge from the interaction of simple components within a system. In algorithmic architecture, emergence often occurs as a result of the interaction between parametric relationships, generative algorithms, and optimization processes. By harnessing emergence, designers can create designs that exhibit qualities of self-organization, adaptability, and resilience.
-
Computational Thinking:
Computational thinking involves approaching design problems with a mindset informed by principles of computation and algorithmic logic. It encompasses skills such as abstraction, decomposition, pattern recognition, and algorithmic problem-solving. In algorithmic architecture, computational thinking enables students to break down complex design problems into manageable components, identify algorithmic patterns and structures, and develop innovative design solutions using computational tools and techniques.
Case Studies: Exploring Real-World Applications:
Real-world case studies serve as invaluable sources of inspiration and insight for students navigating the complexities of algorithmic architecture. By examining how computational design principles are applied in practice, students can gain a deeper understanding of the potential and challenges of algorithmic approaches within the architectural context. Let's delve into some compelling case studies that showcase the diverse applications of algorithmic architecture:
-
The Guggenheim Museum Bilbao: Designed by Frank Gehry, the Guggenheim Museum Bilbao is an iconic example of algorithmic architecture in action. Gehry utilized computational algorithms to generate the museum's distinctive curvilinear forms, which were optimized for structural integrity and aesthetic impact. By leveraging advanced parametric modeling techniques, Gehry was able to translate his visionary design concepts into built reality, creating a landmark structure that has become synonymous with contemporary architecture.
-
Beijing National Stadium (Bird's Nest): Designed for the 2008 Beijing Olympics by architects Herzog & de Meuron and structural engineers Arup, the Beijing National Stadium, also known as the Bird's Nest, is a triumph of algorithmic design. The stadium's intricate lattice structure was conceived using generative algorithms, which optimized the placement of steel members to achieve both structural stability and visual elegance. Through the use of advanced computational tools, the design team was able to realize a highly complex and iconic architectural form that captured the world's imagination.
-
Sagrada Família: Antoni Gaudí's masterpiece, the Sagrada Família in Barcelona, exemplifies the fusion of algorithmic architecture with organic forms inspired by nature. Gaudí employed innovative computational techniques, such as ruled surface generation and hyperbolic paraboloid structures, to create the cathedral's awe-inspiring architecture. By drawing inspiration from natural geometries and employing algorithmic principles, Gaudí crafted a building that transcends traditional architectural conventions, embodying a harmony between technology and nature.
-
The Heydar Aliyev Center: Designed by architect Zaha Hadid, the Heydar Aliyev Center in Baku, Azerbaijan, showcases the fluid and dynamic possibilities of algorithmic architecture. Hadid's design for the center was driven by parametric modeling techniques, which enabled the creation of sweeping, undulating forms that seamlessly blend interior and exterior spaces. Through the use of computational algorithms, Hadid was able to realize a vision of architectural fluidity and innovation, transforming the built environment into a canvas for expression and movement.
-
The V&A Dundee Museum: Designed by Japanese architect Kengo Kuma, the V&A Dundee Museum is a striking example of how computational design can be used to create buildings that respond to their cultural and environmental contexts. Kuma employed parametric modeling techniques to optimize the museum's faceted facade, which was inspired by the rugged cliffs of Scotland's coastline. By leveraging computational algorithms, Kuma was able to create a visually stunning and contextually sensitive architectural landmark that celebrates the region's heritage and identity.
Conclusion:
In the dynamic and ever-evolving landscape of architecture and design, algorithmic architecture stands as a beacon of innovation and creativity, offering students a pathway to explore the boundless possibilities of computational design. Through the lens of Rhino and Grasshopper, students have embarked on a journey of discovery, navigating the intricate terrain of parametric modeling, generative algorithms, optimization techniques, and data-driven design principles.
As we reflect on the insights and experiences shared in this exploration, it becomes evident that algorithmic architecture represents more than just a technological advancement—it embodies a paradigm shift in the way we conceive, create, and interact with the built environment. From the iconic curves of the Guggenheim Museum Bilbao to the dynamic forms of the Beijing National Stadium, algorithmic architecture has reshaped the architectural landscape, pushing the boundaries of form, function, and expression.
Through the examination of real-world case studies, students have gained a deeper understanding of the practical applications of algorithmic architecture, witnessing firsthand how computational design principles are translated into built reality. From Frank Gehry's pioneering use of parametric modeling to Antoni Gaudí's organic geometries inspired by nature, each case study offers a glimpse into the transformative power of algorithmic approaches in shaping the architectural zeitgeist.
As students prepare to embark on their own algorithmic architecture assignments, they are equipped with a toolkit of knowledge, skills, and inspiration to guide their exploration. Armed with the foundational concepts of parametric modeling, generative algorithms, optimization techniques, and data-driven design, students are poised to push the boundaries of innovation and creativity in their own design endeavors.
In the spirit of continued inquiry and discovery, students are encouraged to embrace a mindset of curiosity, experimentation, and collaboration as they navigate the complexities of algorithmic architecture. By immersing themselves in the principles and practices of computational design, students can unlock new avenues of exploration, challenge conventional design paradigms, and redefine the possibilities of architectural expression.
As we conclude this exploration of algorithmic architecture within Rhino for assignments, we are reminded that the journey is just beginning. The future of architecture lies in the hands of the next generation of designers, innovators, and thinkers who will harness the transformative power of algorithmic approaches to shape the built environment for generations to come. With Rhino as their canvas and Grasshopper as their brush, students are poised to embark on a journey of discovery, creativity, and innovation that will leave an indelible mark on the architectural landscape.